Preface
This pamphlet is organized into two parts:
Part I provides the reader with an overview of the scientific basis of climate change and its predicted impacts on human populations and natural ecosystems, so they themselves can make an informed opinion of its significance to their own lives and the lives of all current and future generations.
Part II describes the political context within which this process is unfolding. I share my vision of what steps may be necessary to preserve the modern world as we know it to be in the year 2024 – one that is far too fragile to withstand the magnitude of the coming changes if we fail to respond accordingly.
Citations are provided at the end of this pamphlet to offer relevant background material and to substantiate my arguments against climate denialism.
Ultimately, the question is not whether climate change is real; it is whether we, as a global society, are prepared to confront humanity’s enormous collective influence on the environment. Will we enact, implement, and engineer the solutions demanded of us before the crisis escalates beyond our ability to mitigate it, or are we destined to become climate refugees, roaming the planet in search of basic necessities like food and water?
I trust the average American citizen has not only the capacity to understand the urgency of this issue, but will recognize that addressing the existential risks posed by climate change is not merely a practical necessity—it is a moral imperative.
Part I
Introduction to Key Concepts and Ideas
Question: What is “climate” and how is it different than weather?
Answer: Climate, in its simplest form, can be thought of as the “statistics of weather.” In this purely descriptive sense, referring to “the climate” of a given region typically means discussing its predominant weather patterns and corresponding averages of temperature, humidity, and precipitation over seasons, decades, or even centuries. Within this context, weather can be viewed as an “expression” of climate: temporary atmospheric phenomena occurring locally over short periods of time (rain, snow, wind, etc.) [1, 2]
However, in a broader sense, climate is only one aspect of the Earth climate system (ECS), a chaotic system constantly being forced out of thermodynamic equilibrium, influenced by the gradually evolving interaction of the sun’s radiation with the Earth’s shifting geography, oceanic circulation, and atmospheric chemistry, among other factors. This understanding emphasizes that climate is both a reflection of long-term weather patterns and a dynamic component of a complex and ever-changing global system.
Question: What is climate change?
Answer: Climate change is a long-term change in the average weather patterns that have come to define Earth’s local, regional and global climates. [3]
Question: What is global warming?
Answer: Global warming is a long-term trend of rising global average temperatures, specifically the combined 30-year average of air temperatures over land and sea surface temperatures, known as the global mean surface temperature (GMST). [4]
Question: How is global warming measured?
Answer: Direct measurements of the Earth’s air and ocean temperatures extend as far back as 250 years for some locations, although it was not until the late 1800s that a sufficient number of observing stations have been able to produce reasonable GMST estimates. [5]
Furthermore, because the GMST represents only a surface manifestation of the Earth’s heat energy accumulation, arguably the most important metric for global warming is the Earth’s energy imbalance (EEI), which calculates the net difference between the total amount of solar radiation absorbed by Earth and the amount of energy emitted back into space (primarily as outgoing thermal radiation.)
Note: Satellite measurements running continuously since 2001 have shown the Earth’s energy imbalance is rising at an alarming rate, having doubled over this period. [6]
Question: What causes global warming?
Compared to all other human and natural factors, the concentration of carbon dioxide (CO2) and other trace gases in the atmosphere have the greatest overall impact on the Earth energy imbalance and therefore global warming. [7, 8]
Question: How does CO2 influence global temperature?
Answer: The Greenhouse (GH) effect describes both:
- The warming and cooling effects of greenhouse gases (GHGs) which absorb and/or reflect incoming solar radiation.
- The warming effect of GHGs which absorb Earth’s thermal radiation, impeding the loss of heat to space.
Ultimately, only a portion of the sun’s total incoming solar radiation is absorbed by Earth, and less still is reemitted back into space as thermal radiation due to the presence of GHGs in the atmosphere. This translates to roughly 12% of all of solar energy returning back to space, even though 17% is converted to thermal energy. [9]
Note: This 5% of total solar energy trapped by GHGs is responsible for sustaining life on Earth; without it, Earth’s surface temperature would be 32 °F below freezing, or about 59 °F (33 °C) colder than what it is today. [10]
Question: How do we know humans are responsible for the atmospheric CO2 causing global warming?
Answer: Human activity leaves a distinct isotopic fingerprint on the CO₂ in the atmosphere, confirming that the rise is largely driven by burning fossil fuels and not natural phenomena. [11]
Question: What natural factors are responsible for global warming?
Answer: Natural factors influencing Earth’s climate system include solar output (irradiance), the Earth’s orbit (Milankovitch cycles), ocean circulation, and the reflectivity of the Earth’s surface (albedo). [8]
Question: How are climate change and global warming related?
Answer: The remaining 5% of solar energy trapped by greenhouse gases (GHGs) in the atmosphere is converted into various forms of energy, including sensible heat (related to temperature), latent energy (associated with phase changes in water, such as evaporation and condensation), potential energy (related to gravity and elevation), and kinetic energy (related to movement). The climate system responds to this excess heat by increasing atmospheric and oceanic circulation in an attempt to restore thermodynamic equilibrium.
Most of Earth’s radiative energy is absorbed at the surface, where it intensifies the hydrological (water) cycle by increasing evaporation and atmospheric moisture content. Regions with minimal rainfall experience more pronounced drying and subsequent heating; conversely, the evaporated moisture, transported by atmospheric currents, converges into storms and frontal systems, leading to increased precipitation rates in other regions. Certain weather systems, such as hurricanes and thunderstorms, are further intensified by the release of latent heat.
Finally, as warmer, moister air becomes more buoyant, it rises and expands in the lower pressure of the upper atmosphere. This cooling air then spreads out and may descend thousands of kilometers away, warming again as it compresses under higher pressures, ultimately redistributing energy across the globe. This dynamic redistribution of energy and moisture contributes to the broader patterns of climate change, with global warming serving as a central driver. [12]
With Key Concepts and Ideas defined, let’s look at some numbers.
Before delving into how GHGs are accelerating global warming and magnifying future climate change, it’s crucial to grasp the sheer volume of carbon dioxide being produced annually on a global scale.
In 2022, 5.05 billion metric tons (Bmt) of CO2 were emitted by the U.S. [13]
While the sources of these emissions vary across fuel type and industrial application, one could imagine the same amount of CO2 being released from a daily combustion of 163 Salesforce Towers filled to the brim with pure 100% octane gasoline. [14]
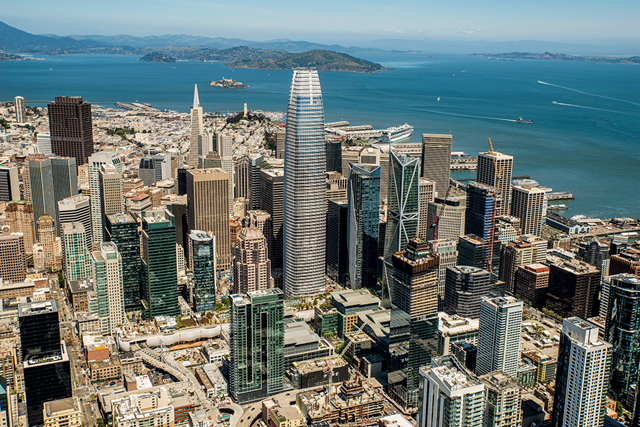
Furthermore, because CO2 is not the only GHG being pumped into the atmosphere (methane (CH4) and nitrous oxide (N₂O) also impede the loss of heat to space), a more complete summarization of the United States’ impact on the climate system is that in 2022, U.S. greenhouse gas emissions totaled some 6,343 million metric tons of carbon dioxide equivalents “CO2e.” [15]
Note: The unit “CO2e” represents the amount of a GHG whose atmospheric impact has been standardized to that of one unit mass of carbon dioxide, based on its global warming potential (GWP). [16]
Using this standardized metric, we can imagine the total U.S. greenhouse gas emissions for the year 2022 having a similar GWP of approximately 174 Salesforce Tower equivalents of crude oil getting torched every day. [17, 18]
And this is just the United States; China and India are combusting 392 and 97 Salesforce Tower equivalents of crude oil on a daily basis, respectively. [19]
Now let’s add some context to our numbers.
Parts per million (ppm) is a dimensionless quantity used to measure the concentration of a substance in a mixture, such as how many molecules of a particular gas, like CO2, are present per million molecules of air.
ppm = (number of molecules of X / total number of molecules in the mixture) x 106
Example: The most abundant gas in Earth’s atmosphere, nitrogen (N2), makes up nearly 78% of the total amount of molecules present in the air we breathe.
N2 ppm = (78/100) x 106 = 780,000 ppm
Since the beginning of the industrial revolution around 1750 A.D., the world has emitted an estimated 1.63 trillion tonnes of CO₂ (1,600 gigatonnes, or GtCO₂). This has led to nearly a 50% increase in atmospheric CO₂ concentrations, from 280 ppm to 419 ppm by 2023. [20, 21]
Note: Considering that 3.67 tonnes of CO₂ equates to one tonne of carbon, the 1.63 trillion tonnes of CO₂ released corresponds to approximately 445 gigatonnes of carbon (GtC), an amount surpassing the 400 GtC estimated to be stored in all of the world’s forests today. [22]
For context, across all the cycles between ice ages and warmer periods over the past million years, atmospheric CO₂ levels never exceeded 300 ppm. [23]
The Keeling Curve, which records atmospheric CO₂ levels through direct observations at the Mauna Loa Observatory in Hawaii, illustrates that in the last 60 years, CO₂ concentrations have far surpassed this historical range. These levels are now increasing at an annual rate three times higher than at the start of the record, reaching 2.4 ppm per year during the 2010s. Additionally, it shows that more than half of all cumulative global CO₂ emissions have occurred since 1990. [23, 24]
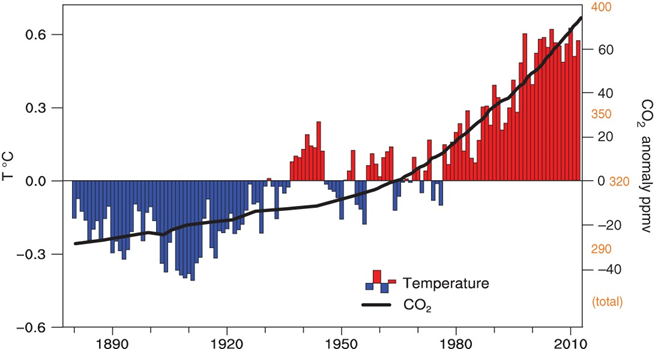
A Brief Note on the Metric System
It seems appropriate at this time to make the argument that the American public’s disconnect regarding humanity’s undeniable impact on modern-day climate change (a rejection of the scientific consensus not shared throughout the rest of the western world) is due in part to our reliance on the imperial system of measurement. I argue that this antiquated unit of measurement limits the American public from readily accepting the clear scientific evidence of anthropogenic (“human-caused”) global warming, especially when international agreements and scientific reports are defined in terms unappreciable to the average American.
(There is of course the imposing presence of the oil and gas lobby, along with a militaristic foreign policy focused on controlling the scarcity of global energy markets. See later: Oil is Power.)
Nevertheless, because this document is aimed towards an American audience, temperature values will be listed in both degrees Fahrenheit (°F) and Celsius (°C).
As an additional side note, the scalability of the metric system allows for a more straightforward conversion between the intuitive and the planetary units of measurement. The 4.6 billion metric tons (Bmt) of CO2 emitted by the U.S in 2020 can be easily converted to 4.6 petagrams (Pt) or 4.6×1015 grams, a figure that is simply more approachable than trying to imagine what 10.1 trillion pounds of CO2might look like.
Ok, so what’s the point?
As previously stated, the greatest contributing factor to present-day global warming is the steadily increasing concentration of CO2 and other GHGs in the atmosphere impeding the loss of heat to space.
But how does global warming affect you? Should you be concerned?
To fully grasp the urgency of addressing global warming, we must first understand the science behind it. Specifically, we need to explore the concept of climate sensitivity—the measure of how responsive Earth’s temperature is to increasing levels of GHGs. This understanding is crucial for evaluating the resilience of our planet’s life-support systems and determining how well humanity can adapt to future changes. (See:The obvious question: Should climate change be taken seriously?) [25]
To begin, a forcing agent is anything whichalters the balance of incoming and outgoing energy at the top of the Earth’s atmosphere (TOA), measured in watts per meter2 (w/m2).
Note: We know GHGs are responsible for global warming because the troposphere (the lowest region of the atmosphere) is warming, while the TOA (stratosphere) is cooling. This cooling is caused by the absorption of heat near the Earth’s surface, which prevents it from escaping into space.
The process by which GHGs absorb energy and remit a portion as heat in the lower atmosphere is known as radiative forcing. Beyond their absorptive properties (radiative efficiency), GHGs are also distinguished by how long they stay in the atmosphere (lifetime). [26, 16]
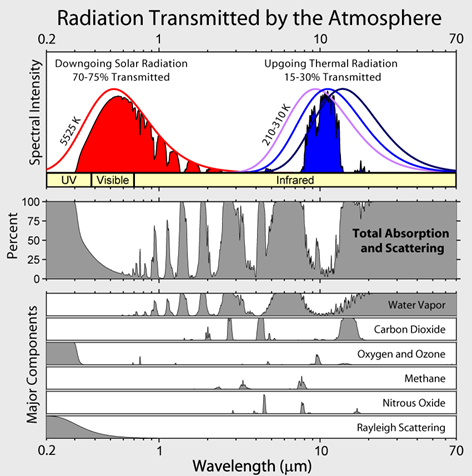
How GHGs influence both the magnitude and rate of future global warming is highly complex for a variety of reasons, including uncertainties in their future atmospheric concentrations, the presence of non-linear amplifying feedbacks, and the inherently chaotic nature of the climate system, all of which make it difficult to distinguish the climate system’s response to various forcings from its natural variability.
- Addressing the unknown future of GHG emissions:
- Global Climate Models (GCMs) are key tools for predicting the response of the climate system to a variety of natural and anthropogenic forcings by conducting thousands of simulations of the Earth’s physical and chemical interactions between the atmosphere, land, and the oceans. [27]
Note: Across all RCPs there exists the high likelihood of breaching 3.6 °F (2 °Celsius / °Kelvin) of global warming by the end of this century, and while there is still considerable debate about the possibility of achieving the “high-emission” scenario RCP8.5 (due to the enormous amounts of CO2 that would need to be released and the uncertainty of near-term feedback), such high-end possibilities cannot be ruled out, primarily due to uncertainty of feedbacks.
- Representative Concentration Pathways (RCPs) are hypothetical emission scenarios compiled by climate scientists and researchers to explore future trajectories of GHG emissions, which are then used as inputs for climate models to predict future climate conditions based on different levels of forcing.
For example: RCP8.5 represents a high-emission scenario, originally designed to model a future with limited adoption of renewable energy, resulting in an additional 8.5 W/m² of radiative forcing by the end of the century. Although this scenario is often labeled “business as usual,” many climate scientists and energy economists question its likelihood, as the rapid cost decline of renewable technologies has made a future dominated by fossil fuels less probable. Nonetheless, RCP8.5 remains a valuable tool for examining worst-case outcomes. [28]
- Shared Socioeconomic Pathways (SSPs) are widely used in current climate research to explore how global socio-economic trends can influence future greenhouse gas emissions and climate change impacts. These pathways account for a broad range of factors, including economic growth, energy consumption, land use, and social equity, providing a more comprehensive framework for understanding how societal development might shape climate outcomes.
For example: SSP2, or the “Middle of the Road” scenario, describes a world where social, economic, and technological trends generally follow historical patterns. Development and income growth occur unevenly, with some countries making significant progress while others lag behind. Global and national institutions aim to achieve sustainable development goals but make only gradual progress. Environmental degradation persists, though there are modest improvements, and overall resource and energy use become less intensive.
- Feedbacks contribute to an overall increase and acceleration of global warming beyond the initial forcing, and are generally classified as either fast or slow feedbacks based on their response times, or the time horizon over which they significantly affect the climate:
- Fast feedbacks respond to changes in the climate system on relatively short timescales, typically within a few years to decades.One significant fast feedback is water vapor, which accounts for about half of the Earth’s greenhouse effect, followed by clouds with approximately 25% (clouds can both reflect and trap heat), and CO2 with about 20%. Water vapor, being condensable (capable of changing from a gas to a liquid), is unique among greenhouse gases in that its concentration increases in response to global warming, thereby amplifying the overall forcing. Atmospheric water vapor varies seasonally but averages around 3,809 ppm annually, making it about nine times more concentrated than CO2 in the atmosphere. [29, 30, 31]
Note: As a quick aside, the same nitrogen that makes up 78% of the atmosphere captures no heat at all.
- Slow feedbacks operate over longer timescales, ranging from decades to centuries or millennia. They often involve large-scale processes in the Earth system that take longer to respond to initial changes but can have profound long-term impacts. Examples of slow feedbacks include melting polar ice caps and declining sea ice formation. Since sea ice reflects more incoming solar energy than the ocean surface, a reduction in total area leads to an acceleration of the overall warming.
- Not all feedbacks act simultaneously; some require that the climate system is sufficiently forced towards a new equilibrium. Once these global temperature thresholds, or tipping points, are crossed, however, the pace of climate change accelerates significantly and becomes irreversible within human timescales, making adaptation more challenging and the long-term consequences inescapable. This accelerating change is further compounded by the inertia of the climate system, which causes a delayed response to current forcing, meaning that if global GHG emissions were to cease today entirely, there would still be a 10-year lag before the maximum warming effect is realized. Therefore, past emissions are already ‘baked in’ and future warming should be anticipated over the next decade before the climate system can stabilize and progress at the ‘baseline’ rate. [32, 33]
But what even is baseline climate change?
Putting things in perspective
Climate scientists rely on the fossil record of prior climates, known as proxy data, to produce estimates of prehistoric climates in a field of study called paleoclimatology. Examples of paleoclimate proxy data include coral reefs, tree rings, ice cores, and lake sediments.
This body of evidence collectively points to a million-year history of recurring Ice Ages and Warm Periods. The last Ice Age peaked around 20,000 years ago during the Last Glacial Maximum (LGM), with global temperatures approximately 11°F (6°C) colder than today and much of North America covered by ice up to a mile thick.
This was preceded by the most recent interglacial period, known the Eemian, which occurred around 125,000 years ago. Climate reconstructions from this point in the Earth’s history to the present indicate that the warmest 200-year-long interval prior to industrialization happened approximately 6,500 years ago, where global temperatures are estimated to have been 1.26 °F (0.7 °C) warmer than the 19th century. [34, 35]
Across these successive climate states, Earth’s ecosystems experienced profound transformations. Temperature shifts, changes in precipitation patterns, and fluctuations in sea levels reshaped vegetation cover, species distributions, and entire biomes. These natural changes illustrate Earth’s remarkable ability to adapt to varying climate conditions over time. [36]
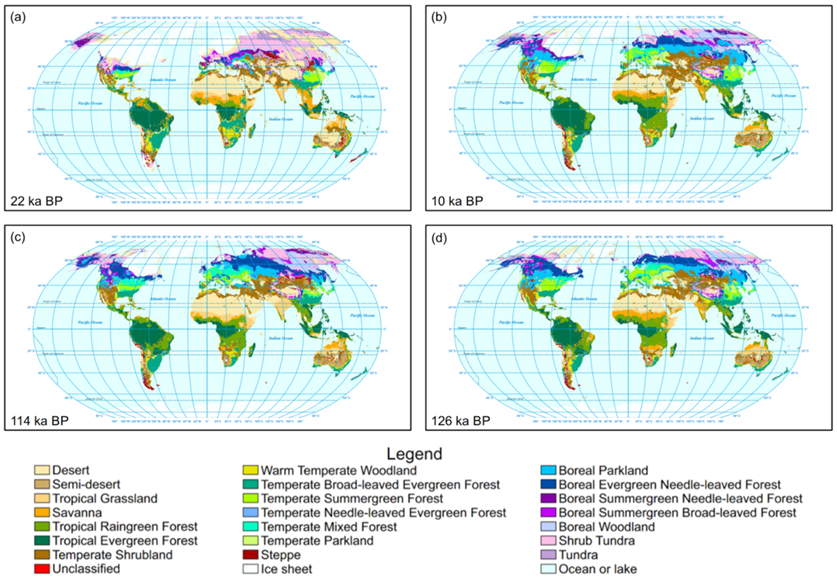
Looking further back into Earth’s geological history, our current level of warming seems even more trivial by comparison. For instance, during the Cambrian Period, which occurred roughly 542 to 485 million years ago, atmospheric CO₂ levels may have been 20 times higher than today, with global temperatures estimated to be as much as 18°F (10°C) warmer.
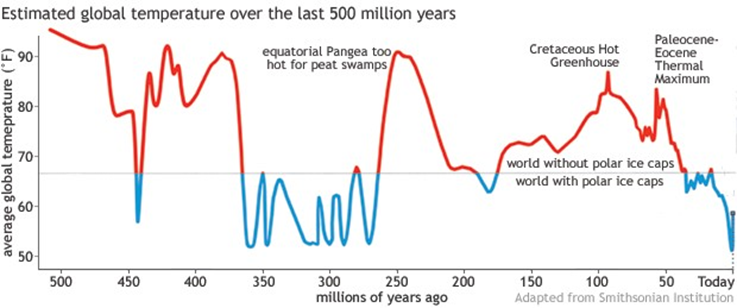
In light of the fact that Earth and its various lifeforms were able to persist through such conditions to the present day, we should specificy that it is the rate of warming, not the absolute temperature increase alone, which will determine the severity of future changes, as present-day global temperatures are rising around 10 times faster than any climate pattern seen in the last 65 million years. This rapid pace of change poses new challenges, as ecosystems, and even human societies, may not have time to adapt. [37, 38]
But even then, what kind of man-made calamity, if any, could emerge from a seemingly modest 3.6 °F (2 °C) rise in the GMST?
A Brief Note on Scientific Communication & the Semantics of Suffering
Contrary to popular belief, most climate scientists adopt a highly conservative approach in their projections of future climate change, often framing potentially catastrophic outcomes within statistically sound ranges to avoid being perceived as making controversial claims. Additionally, the objective tone they employ is intentionally devoid of emotional gravity, which can obscure both the urgency of the situation and the true human toll of the crisis. To break away from this sanitized jargon and inject some empathy into the discussion, I will refer to these projected impacts as real-world consequences (RWCs) to emphasize their tangible effects unfolding in real time. Finally, without international cooperation to significantly reduce CO₂ emissions, the most perilous outcomes of this crisis should not be dismissed as mere climate alarmism; they are, in fact, the most likely scenarios.
The obvious question: Should climate change be taken seriously?
Before answering that, let’s consider this: what truly matters to you? Do you care about anything?
Perhaps you’re concerned about:
- Elementary school students using gender neutral bathrooms to hide from active shooters?
- American pharmaceutical jobs being outsourced to Latin American cartels?
- Hungry polar bears and poor ski seasons?
- Whether humanity can survive beyond the next century?
Considering the full spectrum of risks and dangers preoccupying the worries of the average American, I assert that:
- Future climate change will have real-world consequences of truly existential proportions.
- Mitigating future climate change should be our nation’s top priority.
My position is grounded in the following RCWs expected to unfold in the absence of a global, coordinated effort to reduce GHG emissions to zero.
RWC #1: Unbearable Temperatures will leave Major Population Centers Uninhabitable
When it comes to increasing temperature extremes, what is bearable, and what is simply beyond the limit of human survival?
The heat index combines air temperature and relative humidity to produce a standardized metric of how humans perceive heat (or the apparent temperature) across different climates. Building upon this, the Wet-bulb globe temperature (WBGT) accounts for all four major environmental heat factors: air temperature, humidity, radiant heat, and air movement. Metrics like these help describe conditions capable of inducing heat stress, or the body’s inability to release excess heat. The body’s natural mechanism for reducing internal temperature relies on perspiration and the evaporative cooling of sweat. However, as temperatures rise and relative humidity approaches 100%, this cooling effect diminishes until it is entirely impossible to dissipate metabolic heat. [39, 40]
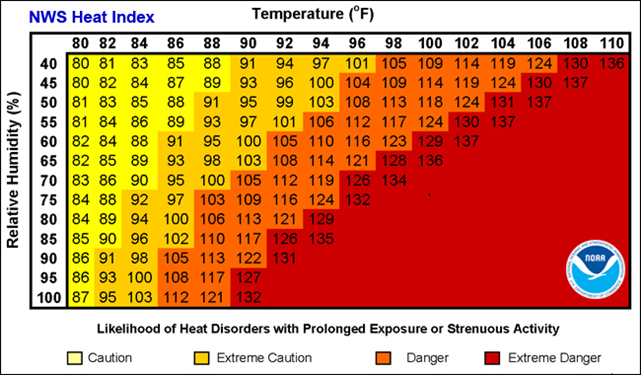
Whereas increasing temperature projections may appear rather ordinary (~3–6°F by the end of the 21st century) by themselves, in conjunction with an elevated relative humidity the likelihood of high-mortality extreme heat events is magnified greatly. In fact, global warming has already increased the severity of heat waves in places like Texas by as much as ~8–11°F (~5–6°C), on average, during June, July, and August (JJA), compared to the pre-industrial baseline (1850-1859). [41]
Globally, the current peak heat stress, as quantified by the wet-bulb temperature (TW)—which ignores direct sun exposure—never exceeds 88°F (31°C). However, under the high-emission scenario RCP8.5, large, densely populated regions will experience seasonal wet-bulb temperatures that put even young, healthy individuals at risk of heat stroke when completing simple daily tasks. [40, 42]
Finally, as a possible sign of things to come, in June and July of 2021, the Pacific Northwest of Canada experienced one of the most extreme regional heat events on record. Air temperatures soared 28–36°F (16–20°C) above normal, with numerous maximum temperature records broken by more than 9°F (5°C). [43]
RWC #2: Droughts Become Longer, More Intense, and Affect Entire Continents
Droughts are generally defined as prolonged deficiencies in precipitation causing water shortages and adverse impacts on vegetation, animals, and people.
In response to global warming, the increase in water vapor in the atmosphere leads to greater evaporation from bodies of water and evapotranspiration from soils and plants. This results in reduced river flows and heightened water stress on ecosystems and agriculture. As this warmer climate favors rain over snow—a notable instance of amplifying feedbacks—a less reliable snowpack contributes to more irregular river flows and more frequent and severe hot-dry events. [44]
The impact on water resources is already critical, with many reservoirs in the West requiring multiple years of above-average precipitation and zero usage to reach full capacity. On May 9, 2024, the Department of the Interior signed a new Record of Decision (ROD) to implement the Lower Basin’s commitment to conserve 3 million acre-feet of water to address critical elevations in Lakes Powell and Mead (the two largest reservoirs in the U.S.) through 2026. Furthermore, the Great Salt Lake is at risk of disappearing completely, which could have devastating consequences for Utah’s public health, environment, and economy. As time progresses, there are fears that even randomly occurring seasons of average to below-average precipitation could trigger droughts in the southwestern U.S. that are more intense than what would be expected from rainfall or snowpack deficits alone. [45, 46, 47, 48]
The vapor pressure deficit (VPD) represents the difference between the actual vapor pressure (the amount of moisture in the air) and the saturation vapor pressure (the maximum moisture the air can hold when saturated). This deficit is known to significantly contribute to tree mortality and soil desiccation. In California’s Central Valley, one of the most agriculturally productive regions in the United States, the number of days with a VPD of ~9 kPa is projected to increase by 70 to 100 days before the end of the century under the high-emission scenario RCP8,5. This VPD equates to a relative humidity of 10% and an air temperature of 114.8°F (46.0°C). [49, 50]
In essence, the concept that droughts are random, temporary episodes of reduced precipitation may soon prove invalid, as the rapid aridification of southwestern U.S. will usher in an era of multidecadal droughts with increased frequency and severity, ultimately rivalling the greatest megadroughts of the last 500 years. [45, 51]
Compared to individual extreme events, compound drought-heatwaves (CDHW) events severely threaten socioecological systems by increasing the risk of larger wildfires, crop failures, and heat-related mortalities. Under the highest emissions scenario, CDHW events are projected to increase by tenfold globally. Furthermore, there is growing evidence that under warming scenarios of 2.7–5.4 °F (1.5–3 °C) above preindustrial levels, the magnitude and extent of droughts, along with the frequency of decade-scale megadroughts, are all likely to increase across most global land areas. [52, 53]
RWC #3: More Extreme Weather and Accelerating Sea Level Rise
Global warming is projected to increase precipitation rates and intensities of tropical cyclones; however, the small magnitude of these changes observed thus far, when compared to natural variability, complicates conclusive attribution. Despite this, some scientists advocate for the addition of a hypothetical Category 6 to the Saffir-Simpson Hurricane Wind Scale. They point out that between 1980 and 2021, all five storms that exceeded the threshold for a new Category 6 occurred within just nine years of that record. This increase is attributed to warming ocean waters, which enhance the available sensible and latent heat energy, thereby raising the thermodynamic potential wind intensity of these storms. Conversely, the decline in the frequency of tropical cyclones in the Northern Hemisphere is much clearer over the 20th century. This trend is explained by the weakening of the Hadley and Walker circulations throughout the century due to global warming. [54, 55, 56]
Global sea level rise (SLR) significantly amplifies the destructive potential of hurricanes by increasing storm surges, particularly in low-lying coastal areas. The repercussions of SLR will profoundly affect coastal communities worldwide for centuries. This phenomenon is primarily driven by thermal expansion of ocean water and the mass loss from glaciers and ice sheets. Currently, the fastest warming occurs in Earth’s polar regions, exemplified by the unprecedented heat wave in March 2022 that impacted East Antarctica, resulting in widespread temperature anomalies of 54–72°F (30°–40°C) across the ice sheet. Various factors contribute to the destabilization of these ice sheets, including increased submarine melting, the loss of floating ice shelves, and the albedo effect of melt ponds. Scientists estimate that an SLR of at least 10.7 ± 2.67 inches (274 ± 68 mm) is already committed for this century, irrespective of future climate scenarios. However, this figure is minimal compared to the catastrophic projections of 9–10 meters of SLR due to the complete collapse of major Antarctic ice shelves by the end of the century. Such a dramatic rise would submerge the low-elevation coastal zone (LECZ)—defined as the contiguous land along the coast below 10 meters in elevation—of countries like China and Bangladesh, which have seen population growth in these areas at nearly twice the national average from 1990 to 2000. In the San Francisco Bay, the risk of flooding is exacerbated by the possibility of more severe droughts, which may result in localized subsidence of coastal land. Projections indicate that a rise of 20 inches in sea levels could inundate buildings worth approximately $17.9 billion in California by 2050, highlighting the need for substantial investments in critical infrastructure, such as coastal sewage and wastewater treatment facilities. [57, 58, 59, 60, 61, 62, 63]
RWC #4: Starvation, Mass Migration, and the Rise of Authoritarian Regimes
While global warming’s destabilizing effects on human populations and ecosystems are increasingly evident, the long-term risks it poses to global stability and human survival remain significantly underexplored. Nevertheless, a growing body of research highlights severe threats to global food security under future warming scenarios. For instance, the probability of simultaneous crop production losses exceeding 10% in any given year across the top four maize-exporting countries—responsible for 87% of global maize exports—rises to 7% with a 2 °C increase (approx. RCP4.5) and escalates to 86% with a 4 °C increase (approx. RCP8.5). At 4 °C of warming, annual agricultural losses are projected to reach around 139 million tons (135.5–142.0), or roughly 14% of current global production—surpassing today’s total maize exports. [64, 65]
The risk of multiple breadbasket failures is linked not only to rising temperatures but also to large-scale fluctuations in the Earth’s atmosphere and oceans, particularly through phenomena known as Rossby waves. These waves, which meander through the mid-latitudes with lengths of about 600 miles (~1,000 km), drive the jet stream’s flow and create persistent high- and low-pressure zones that shape regional weather, are referred to as synoptic waves. Under high-emission scenarios, Rossby waves are projected to nearly double in magnitude by the end of the century. This intensification could lead to more severe heat domes—extended periods of trapped heat within high-pressure systems—over key agricultural areas. When synchronized across continents, these stationary waves could trigger crop failures worldwide, leading to critical food shortages in agriculturally dependent regions. [66, 67]
Historically, human civilization has thrived in a “climatic envelope” with a mean annual temperature (MAT) of around 51.8 to 59 °F (11 °C to 15 °C), where favorable conditions have supported agricultural productivity and population growth from nearly pole to pole. However, under severe climate change scenarios, this “hospitable zone” is projected to shift more dramatically in the next 50 years than over the past three millennia. By 2070, a MAT of 84.2 °F (29.0 °C)—currently typical of the Sahara Desert—could extend from covering only 0.8% of the global land area today to 19%, exposing nearly one-third of the world’s population (around 3.5 billion people) to extreme, often uninhabitable heat. Average temperatures in populated areas are expected to rise by 7.5 °C, or about 2.3 times the global mean increase. This drastic warming of land is attributed to faster land heating compared to oceans and to concentrated population growth in already hot regions. [68]
With living conditions approaching the limits of human tolerance, particularly in the tropics, large-scale forced migration may become unavoidable. Unlike today’s concerns over migrant caravans, future migrations may see entire populations displaced by unbearable heat, humidity, and food shortages. Unfortunately, the regions they flee to will likely be facing their own climate-induced challenges, placing immense strain on infrastructure, resources, and societal stability. This cascade of events could fundamentally reshape the global order, with food insecurity and mass migration potentially paving the way for the rise of authoritarian regimes as governments struggle to preserve stability amidst upheaval. [64]
RCW#5: Mass Extinctions and Ecosystem Collapse
While humanity’s adaptation to a rapidly changing climate remains technically possible—at least for those with sufficient resources—the natural world faces a far graver challenge. Ecosystems will undergo extreme disturbances with little capacity to adjust, given the unprecedented rate of climate change. The consequences will likely include mass extinctions and ecosystem collapse, which, aside from their resource value to human economies, possess intrinsic worth beyond measure.
If anthropogenic emissions align with RCP 2.6, about 33% of the Earth’s terrestrial surface (excluding Greenland and Antarctica) is projected to experience significant ecological shifts affecting the climate’s ability to support distinct plant growth forms. Under the high-emission scenario, RCP 8.5, this fraction jumps to 68%, with temperate, boreal, and polar ecosystems facing the most dramatic changes. [69]
As mentioned earlier, rising atmospheric temperatures enhance the air’s moisture-holding capacity, resulting in somewhat paradoxical conditions where terrestrial landscapes may dry out despite increased precipitation. Generally, arid ecosystems can better withstand these conditions, while plants adapted to wetter climates suffer. However, in drier regions, wildfire risks are greatly amplified. This trend is starkly evident in California, where wildfires burned a combined area in 2020 and 2021 amounting to ten times the annual average since the late 1800s. [70, 71]
Marine ecosystems are also imperiled by global warming, as rising ocean temperatures drive up the metabolic demands of aquatic organisms while simultaneously reducing oxygen solubility. This combination effectively shrinks the ocean’s habitable zones. Even under a scenario where CO₂ levels briefly overshoot before stabilizing due to carbon dioxide removal (CDR) efforts, oxygen-rich waters in the upper 200 meters—where most marine life resides—are projected to decline by 13–29%. Such chemical alterations render the future ocean environment effectively irreversible within human timescales. [72]
Moreover, unlike terrestrial ecosystems, the ocean is also sensitive to the concentration of atmospheric CO2 because of the direct impact it has on ocean acidity as it dissolves over time. Based on business-as-usual emission scenarios, by the end of this century, ocean surface waters could be nearly 150 percent more acidic, resulting in a pH that the oceans haven’t experienced for more than 20 million years. This drastic acidification threatens the survival of marine species, from corals to shellfish, disrupting entire food webs. [73]
One last look
Since the early 1800s, most mammals, birds, and amphibians have seen their ranges diminish significantly, primarily due to urban expansion and agricultural land conversion—human activities that have profoundly reshaped natural habitats. Today, 16% of existing species have lost over half of their natural range. However, anthropogenic climate change has also caused certain species’ ranges to expand, with an estimated 18% now inhabiting new territories as formerly unsuitable vegetation is converted to cropland and pasture. [74]
This shift, however, comes at an immense cost. Range expansion for a few species does not offset the growing number of threatened species or the accelerating rate of range reduction overall. The long-term trajectory of land-use change now encroaches more deeply into tropical regions—areas with the greatest species richness and where smaller average ranges heighten the impact of habitat loss. In these biodiverse regions, even a small reduction in natural habitat triggers disproportionately high relative losses, amplifying the consequences for both local ecosystems and the global web of life.
Taken together, these changes are reminders of a fundamental truth: the environmental challenges we face are cumulative, interconnected, and driven by complex human influences. As we move forward, understanding this broader context underscores the urgent need to protect not only our species but the countless others that share this planet.
Part II
So now what?
Hopefully by this point, you should be convinced that global warming is a critical issue and that it deserves urgent action. The question now is: what can we do? Unfortunately, despite the overwhelming scientific consensus on human-driven climate change, meaningful action has been stalled for decades by the political influence of the oil and gas lobby and their willing collaborators in the American Republican Party. Had we acted globally when these concerns first emerged, gradual changes to our economy and overall way of life would have sufficed. Instead, emissions have accelerated, pushing us toward a tipping point where transformative changes must occur within years—not decades—to avoid destabilizing effects on global food security and livable conditions worldwide.
Oil is Power
So why hasn’t meaningful action been taken? Blame laid solely on the oil and gas lobby, which has known since the 1970s that unchecked fossil fuel production would lead to global warming, would be inadequate. Oil is deeply embedded within global power struggles: it fuels economies, underpins transportation, and is essential to military operations worldwide. Unlike coal or gas, oil’s scarcity maintains power imbalances, making it an invaluable resource for those who benefit from preserving a status quo of controlled access and enforced dependency. [75]
Thus, the global shift toward renewable energy represents a move to a more democratized energy system, challenging the scarcity-based model that reinforces economic and geopolitical exploitation. Consider the strategic interests in the Middle East, where conflicts often intersect with energy security, driven by nations vying for control over oil reserves. The Israeli-Palestinian conflict clearly demonstrates this dynamic, as the Israeli regime receives unconditional support in exchange for U.S. military access to the region.
Lastly, most of the developers of sustainable energy projects are, in fact, oil and gas companies that characterize their investments as a diversification of their portfolios. In reality, they have no serious commitment to renewable energy or anything else that disrupts their core business models. Plans are invariably delayed or canceled. True progress, therefore, must come directly from state-led initiatives, which should be free of conflicting commercial interests.
Achieving the Unprecedented
Rapid advancements in renewable energy technologies provide a technically feasible path forward to mitigate future global warming. However, the task remains daunting: emissions must peak by 2025 and then sharply decline. The central challenge has always been how to foster international cooperation, given that climate risks are felt disproportionately across nations. In essence: who pays for it? For developed nations, severe risks to food security could reverse a century of progress in reducing childhood hunger. Meanwhile, less developed countries, with minimal historical emissions, stand to suffer the most. Should developed countries commit to a greater share of funding the transition? [76]
Regardless of who funds the adaptation and mitigation efforts, the cost of inaction will far exceed these investments. It’s estimated that 2.5–3 °C of global warming could reduce per capita output by 15%–25% by 2100, with reductions over 30% at 4 °C, relative to a world with the relatively stable climate system that has supported humanity for millennia. [77]
Building Effective Political Opposition
First, we must recognize the complicity of both major parties in what amounts to a form of global genocide—a crisis bound to unravel the stability of societies worldwide in the coming decades. By failing to address climate change with the necessary urgency, both parties have effectively guaranteed a future of catastrophic human suffering and irreversible damage to natural ecosystems. Our actions can only serve to limit further harm.
Second, if we are serious about avoiding a future marred by decade-long continental megadroughts, we must build an industrial economy that doesn’t rely on the equivalent of 163 Salesforce Towers filled with 100% octane gasoline being set ablaze every day. This transformation requires carbon-free energy not only in consumer sectors like transportation but also in manufacturing and other industrial processes that support our high standard of living. Put simply, we need the machines that create our clean energy infrastructure to be clean themselves—we must electrify everything. [78]
Moreover, our goals must match the scale of the crisis we face. Should we mobilize a million students to plant trees or mandate an immediate end to driving internal combustion engine (ICE) vehicles, rather than merely phasing out their sale a decade from now? And while lifestyle changes like swapping plastic straws for reusable alternatives hold symbolic value, they aren’t systemic solutions. Large-scale, institutional change is essential for meaningful emissions reduction. How much of the federal budget should support this existential mission? In 2023, China added more solar capacity than the U.S. has in its entire history. [79]
Finally, we must be wary of greenwashing—efforts that claim environmental benefits but ultimately reinforce fossil fuel dependency, such as using carbon capture for oil extraction.
Rethinking Environmentalism
Traditional environmentalism, with its focus on protecting individual species and habitats, is increasingly becoming a delusional proposition in a world likely to experience cataclysmic biodiversity loss on a global scale. We must recognize that large-scale extinctions may be unavoidable, no matter what actions we take.
A “New Environmentalism” must prioritize adaptation and difficult choices, such as balancing renewable energy development with its environmental impacts. This approach requires us to weigh both the immediate costs and long-term benefits of solutions like solar, wind, and even nuclear energy. Although nuclear power has been stigmatized by historical disasters, it remains one of the most potent, reliable, and low-carbon energy sources available today. A commitment to advanced nuclear technologies could provide the stable, base-load power needed to replace fossil fuels without the intermittency issues of solar and wind. For this new environmentalism to succeed, nuclear energy must be reevaluated as a core component of a sustainable clean energy future.
Furthermore, in the near term, we may need to consider emergency measures such as injecting aerosols into the stratosphere to increase the reflectivity of the atmosphere, a process known as Solar Radiation Management (SRM). While SRM may help slow warming temporarily, it cannot replace the urgent need to address emissions directly. To that end, CO₂ must be regulated as the pollutant it is, necessitating a global framework that treats atmospheric stability as a shared resource.
Reducing CO₂ emissions is not only crucial for stabilizing the climate; it also offers immediate public health benefits. Cleaner air results in fewer respiratory diseases like asthma and lung cancer, conditions that are often exacerbated by pollutants from fossil fuels. Research has shown that reducing air pollution leads to fewer premature deaths, a healthier workforce, and significant reductions in healthcare costs. Cutting emissions is not just about preventing environmental catastrophe; it’s about fostering healthier communities and reducing the healthcare burden on society. [80]
Reasons for Hope
Progress continues. On December 5, 2022, U.S. scientists achieved “ignition” in a nuclear fusion reaction, a major milestone toward clean, limitless energy. If fusion becomes commercially viable, it could power the large-scale carbon dioxide removal necessary to undo centuries of emissions. Additionally, the drive for renewable infrastructure is gathering speed, with wind and solar capacity growing globally despite opposition from fossil fuel interests. [81]
Moreover, public perception of climate change has evolved dramatically. While early warnings were often dismissed, there’s now broader awareness. However, misinformation and competing narratives—often fueled by vested interests—still shape perceptions. For some, climate change remains an abstract future threat; for others, it’s an immediate crisis. Notably, recent research from Yale and George Mason University indicates that a growing percentage of voters, including 39% overall, now consider global warming a “very important” issue for their vote in the 2024 election. Bridging this perception gap is essential, as collective public pressure is crucial for meaningful policy changes. Increasingly, society recognizes that addressing climate change isn’t just about environmental preservation but about building a healthier, more resilient future. [82]